<< Return to Main Page
Back: The Science of Plume Effects
Lunar Regolith
Resistance to Deep Cratering
The Moon’s unique regolith was formed by billions of years of impacts, including micrometeoroid impacts. The regolith is very loose in the top few centimeters and generally becomes more compacted (less porous) over the next few tens of centimeters of depth. It is very fine so it is relatively impermeable and very cohesive. The particles are rough and angular so it is also highly frictional. As a result, while rocket exhaust can easily scour away the loose material on the surface, it generally provides inadequate pressure to shear the soil and thereby form a deep crater in any of the identified regimes (BCF, DDS, DGEE), at least for the case of the Lunar Module in equatorial or mid-latitude soil. Larger landers or polar soil may produce different results, and more work is needed to find out.
Photometric Disturbance
Around the landing sites we see a change in the photometric properties of the soil, or the way it reflects sunlight from different angles. For each Apollo landing, the blast effects smoothed the texture of the soil over a radius of about 50 to 100 m. For the smaller robotic spacecraft landings, the area of this photometric disturbance was smaller. It has been hypothesized by Noble and Mendell that the lunar soil is covered by an epiregolith, a fairy-castle structure of fine dust particles tenuously balanced by electrostatic forces in the weak gravity, residing in a thin layer only about 6 dust grains thick. They hypothesized this causes the widespread photometric properties of the lunar surface, how it reflects light back in the direction the light came from than in other directions, like the special coatings on road signs. The photometric properties around the landing sites are different than the other parts of the Moon. Apparently the rocket exhaust disrupted the epiregolith by smoothing it out (knocking down the fairy castles), and apparently the epiregolith around the landing sites has not healed even after 40 years since the Apollo landings. Apparently, the geological processe that sets up the epiregolith is not very fast. It is not known exactly how the blast effects of spacecraft landing caused this smoothing of the epiregolith: by the high velocity gas directly pushing down the fairy castles, or the spray of dust ejecta knocking it down, or the deposition of larger particles closer to the lander changing the particle size distribution in the vicinity, or electrostatics in the aftermath of the charged rocket exhaust having been blown into that region transporting the dust, or some other mechanism. Understanding the physics of this photometric brightening will enable us to interpret the length scale of that effect and therefore provide insight into the nature of the rocket blast effects and how to model them.
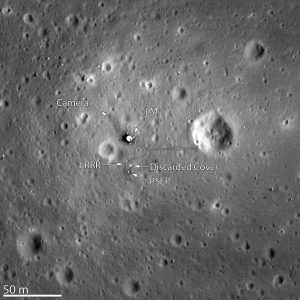
Lunar Polar Regions
There is some evidence that the soil may be less compacted (more porous) at the poles of the Moon than at the equatorial and mid-latitude sites that were previously visited. Metzger and co-workers performed thermal cycling experiments on lunar soil simulant and found that it is an extremely efficient mechanism to densify soil, so other factors being equal the soil should be less dense where there is less thermal cycling — at the poles and especially in the permanently shadowed regions (PSRs).
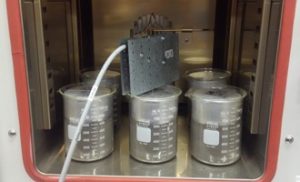

The LCROSS impact in the permanently shadowed Cabeus crater produced radiative emissions and ejecta that both indicate the soil was 70% porous to about 2 or 3 m depth. This is extraordinarily uncompacted, which corroborates the hypothesis. Also, there is a preliminary suggestion that LRO’s Diviner Radiometer data shows the soil has less thermal inertia and may therefore be less compacted toward the poles.
This has important implications for the plume effects. Landing on 2-3 m of 70% porous soil will likely induce bearing capacity failure or other deep cratering mechanisms, making landings far more challenging.
Disturbing the Local Environment
The rocket exhaust strips off the surface layer and injects plume chemicals, including uncombusted fuel, into the regolith. Plume chemicals may freeze into nearby cold traps. Surrounding terrain will be sandblasted. These effects need to be quantified so they can be accounted for in any scientific measurements made by the lander.
Changing the Lunar Atmosphere
Plasma effects
Rocket exhaust is a positively charged plasma, so every landing blows positive charge into the local environment, while the spacecraft is charged negatively. It is unknown what effect this has to the landing site. It is possible this is the explanation for the ~30 sec of lofted dust following each Apollo Lunar Module landing. Our team quantified this dust-lofting in the Apollo landing videos and verified that it cannot be explained by ballistics of the ejecta. It seems more likely to be the result of electrical charging from the plume, less likely to be the result of outgassing of the regolith continuously lofting more dust, although more work is needed to find out.
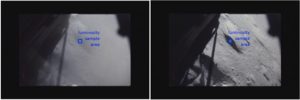
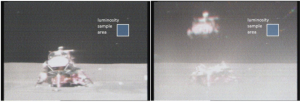
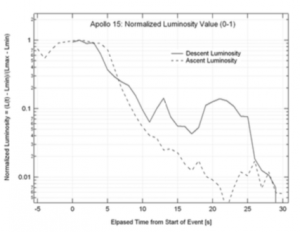
It is also possible that charging by the plume may have a role in the photometric disturbance of the landing site (see above), but there are other possible explanations for that effect so this is just speculation.
This effect might also cause dust to be attracted back to the spacecraft, which could affect solar cells, radiators, and instruments. This (possible) effect has not been noticed in the past missions. A possible exception is the Surveyor 3 mission. Pieces of the Surveyor were brought back to Earth by the Apollo 12 astronauts and were found to be thoroughly coated with dust. This dust was there before the Apollo 12 Lunar Module landed nearby, because our research proved that the sandblasting effect of the Lunar Module actually removed net dust from the Surveyor. It is still unknown why dust so thoroughly coated the Surveyor spacecraft, although our team investigated several hypotheses.
Martian regolith
Asteroid, Phobos & Deimos regolith
There is far too little known about the regolith of asteroids, but this may change with the current missions that are in progress. It is possible that there are geological processes that create layers of regolith with different permeabilities and particle sizes. An example is the thermal cracking that turns large particles into smaller ones, which will only occur in the top of the regolith where the thermal wave exists. The presence of these features in the regolith will have an affect on how a thruster disrupts the regolith. Therefore, we need to be able to analyze these plume-induced disruptions on asteroids and the small martian moons, in every mission where they occur, so we can use those results to help understand the geology.
Next: Modeling Methods to Predict Plume Effects
Back: The Science of Plume Effects
<< Return to Main Page