The Different Regimes of Plume/Soil Effects
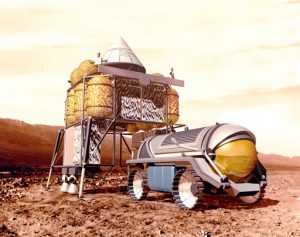
The known regimes (so far) are the following (in the order they were discovered):
- Surface erosion, or scouring, often called Viscous Erosion (VE)
- Diffused gas eruption (DGE)
- Bearing capacity failure (BCF)
- Diffusion-driven shearing (DDS)
- Diffused Gas Explosive Erosion (DGEE)
To this list, we can add some related behaviors:
- Shock impingement splashes
- Initial crater collapse to form the residual crater
- Melting or sublimation of subsurface volatiles
- Etc.
Each regime will be described below.
Viscous Erosion (VE)
Viscous erosion is the main regime during lunar landings. The rocket exhaust strikes the soil, creating a stagnation region (stationary gas) beneath a stand-off shockwave directly under the nozzle. This hot gas expands radially away from the stagnation region, expanding and accelerating as it travels outward into vacuum. As it blows along the surface, it picks up lunar soil particles including dust, sand, gravel, and rocks, accelerating them to high velocity. The astronauts noted the horizon looked hazy during the landings, so we know the dust blew fast enough to go completely over the horizon.
Our team members have accomplished the following research on the VE regime.
- Measured the way the blowing dust distorted the shadows of the Lunar Module, and used that distortion to derive the trajectories of the blowing dust particles. (Immer et al., 2008; 2011)
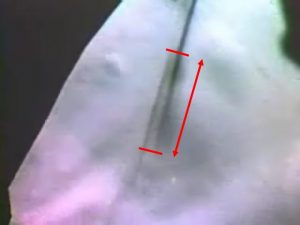
- Performed computer simulations to determine particle trajectories through gas flow fields from several types of flow solvers (including rarefied flow methods), and verified they match what was measured via the Lunar Module shadows. (Lane et al., 2008; 2010)
- Developed highly accurate constitutive equations for lift and drag of particles blowing in the full range of Mach and Knudsen numbers and produced a model the predict ejected particle trajectories. (Lane et al, 2010; Lane and Metzger, 2011)
- Measured blowing rocks that are visible in the Apollo landing videos and confirmed their predicted trajectories match the computer simulations. (Metzger et al, 2011)
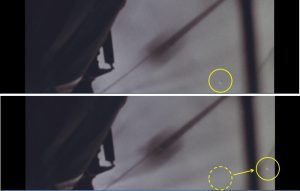
- Calculated the altitudes at which blowing dust begins for different sized landers (Lane et al., 2012)
- Performed experiments of regolith erosion in the continuum, subsonic regime as the basis for the more challenging cases, successfully deriving an erosion rate equation relevant to all variables. It turned out that erosion rate is proportional to momentum flux in the experiment, and inversely proportional to sand grain diameter and sand grain density. (Metzger et al., 2009a; 2009b; 2010)
- Performed experiments of regolith erosion in reduced and increased gravity, deriving the gravity relationship and finding evidence of a cohesive relationship that needs further research. Determined that for non-cohesive cases, erosion rate is inversely proportional to gravity. (Metzger et al., 2009b; 2010)
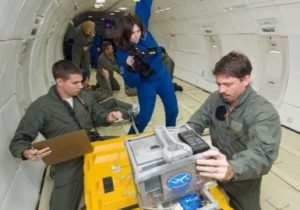
- Performed experiments of regolith erosion for rarefied flow, discovering that erosion rate is faster than predicted for the continuum scaling laws, and derived an approximate, purely-empirical erosion rate equation. (Metzger et al., 2016)
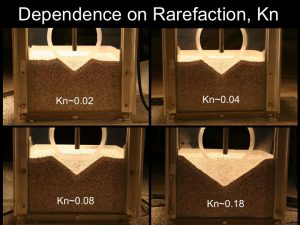
- Measured optical density of the dust in the Apollo landing videos to estimate erosion rate in an actual lunar case. Because the Lunar Module used film cameras with film that is no longer manufactured and is unavailable for brightness calibration, only a limited set of measurements could be made from the videos. (Immer et al., 2008; 2011)
- Created a new statistical method to analyze blowing dust in the Apollo landing videos to create a better measure of erosion rate, and successfully derived an equation relating erosion rate to plume shear stress (Lane and Metzger, 2014a; 2014b)
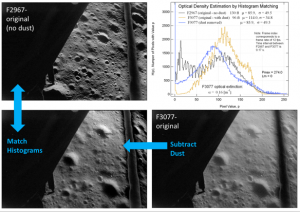
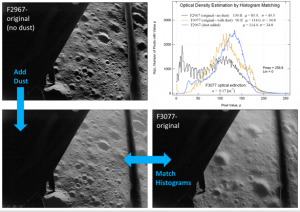
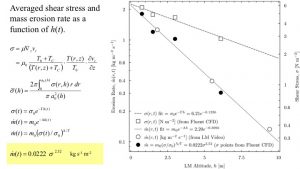
- Analyzed the damage to the Surveyor 3 spacecraft, counting the amount and size of sand grains that struck its surface and the amount of dust scouring, and compared to other predictions of erosion rate; determined the Surveyor was underneath the main sheet of ejecta since it was down in a crater, so damage was 1000 times less than if it had been in the direct spray. (Immer et al., 2011b)
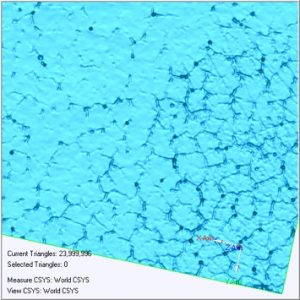
- Worked with several software companies to advance the state-of-the-art in modeling gas interactions with regolith, including supersonic and rarefied flow.
- Thoroughly analyzed and classified the phenomenology of the Apollo landing videos and the modified terrain beneath the lander, and compared to the phenomenology of lunar analogy plume tests. (Metzger et al., 2011)
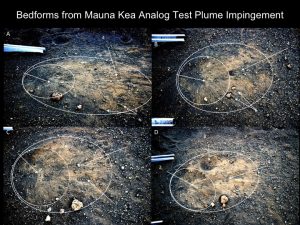
- Developed a modeling method that incorporates all the above results, and applied it to the size range of commercial lunar landers, making predictions of ejecta sizes, velocities, and impact angles as a function of distance from the landing site, integrated over the descent trajectory of the lander (Metzger and Lane, unpublished)
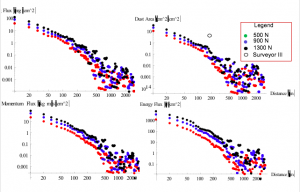
- Used the above results to write NASA’s guidelines for protecting the heritage sites on the Moon. (NASA, 2011)
- Applied theory to the Asteroid Return Mission pulling a boulder off an asteroid where thrusters may be used in proximity to the regolith. (Metzger, 2014b)
Diffused Gas Eruption (DGE)
Diffused Gas Eruption was discovered as a regime by Ron Scott and Hon-Ko in 1968. The idea is that gas diffuses into the soil between the grains of sand and dust, building up pressure to match the pressure of the rocket exhaust on top of the soil. Then, it can erupt in two different ways. One way is that it diffuses laterally under the surface of the soil, building up pressure away from the centerline of the rocket. This creates a force imbalance that makes the soil erupt. The second way is that after the rocket engine shuts off, the sudden loss of pressure no longer balances the pressure under the surface, so soil erupts on the centerline of the engine toward the bottom of the spacecraft.
There is no visual evidence that this occurred in the Apollo landings. Metzger wrote software with high fidelity algorithms of gas diffusion including rarefied (Knudsen), transitional, and continuum (Darcy) diffusion as a function of regolith porosity and other parameters. The simulations showed that gas under a Lunar Module landing will permeate no more than a few centimeters, but that is the same depth over which the loose material is scoured away by VE, leaving more compacted material that resists both permeation and eruption. The pressure gradients are so gradual under the rocket engine in lunar vacuum that the very limited extent of lateral diffusion cannot set up large pressure gradients to cause toroidal eruption.
It also seems unlike DGE will be a significant factor in large human-class Mars landings, because deep cratering (BCF, etc.) will dominate.
The places where DGE may be important are on small bodies such as the Martian moons or asteroids. Chambers at UCF is performing experiments and developing computer simulations to investigate further. Preliminary results show that DGE will happen under the thrusters.
In related work, Metzger wrote software to simulate DGE under the OSIRIS-REx sampling mechanism, which uses gas to scour regolith into itself. New equations had to be developed because the large pressure differences would cause supersonic flow in the subsurface where inertia of the gas cannot be neglected Metzger’s analysis shows that the gas will be driven deep into the subsurface of the asteroid and, depending on the regolith’s porosity under the surface, could blow out a significantly large quantity at the spacecraft. (Metzger, 2014a)
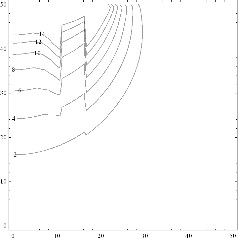
Bearing Capacity Failure (BCF)
In geotechnical engineering, the soil’s ability to hold up a building is its bearing capacity. If too much load is placed on the soil, it will result in bearing capacity failure. Similarly, if a rocket exhaust pushes down with more stagnation pressure than the soil’s bearing capacity, it will push the soil down into a depression, i.e., a crater. This concept was originally studied by Alexander et al. (1966). Researchers on our team discovered several crucial corrections to that theory.
First, the research in Alexander et al. included firing rockets into sand then measuring the resulting crater. What we found is that the resulting crater is just a residual crater that formed from the collapse of the initial crater due to the rocket exhaust, and there is no similarity in their shapes. The residual crater is typically a cone at the angle of repose of the sand. The initial crater formed by BCF is actually a long, narrow cylinder. We found this by performing cratering experiments against a polycarbonate viewing window so we could record the crater with high-speed cameras while the rocket is firing. (Metzger et al., 2009a)
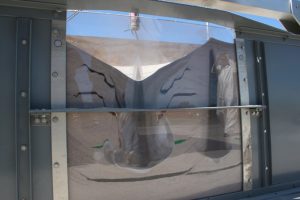
Second, our team discovered a crucial error in the theory by Alexander, at al. The theory assumed that the rocket exhaust diffuses into the soil causing it to loosen, while at the same time the stagnation pressure pushes down on the surface to form the crater. However, if the pressure under the surface were equilibrated with the pressure above the surface, then there would be no surface force to push the soil down. As we explored this, we discovered a new regime, Diffusion Driven Shearing (discussed below). BCF occurs when the jet pushes the soil down much faster than the pressures can equilibrate. DDS occurs when the subsurface pressure develops faster than the soil can be pushed down. (Metzger et al., 2009c)
Third, we found that the depth of these initial craters are far deeper than predicted by the Alexander et al. theory, sometimes by an order of magnitude. The Alexander et al. theory apparently leaves out some of the physics, and it assumes a shearing geometry that is more resistive to subsurface shear than what actually occurs in the soil. More work is needed to develop an accurate theory of the subsurface deformations. (Metzger et al., 2009c)
Fourth, we found that the properties of the soil actually have very little effect on the depth of the initial crater, at least for the cases in our experiments. For one test, we used Mars soil simulant in two very different porosities and mechanical strengths. We found that the rocket exhaust created almost exactly the same size crater in each case. The results suggests the crater depth is a function of the jet length, which is a property of the propulsion system and the ambient atmosphere, not a function of the soil. The crater grew about twice as fast in the case with weaker soil, but it was still just a couple seconds in each case. (Immer and Metzger, 2010)
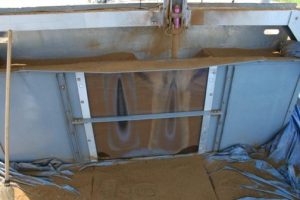
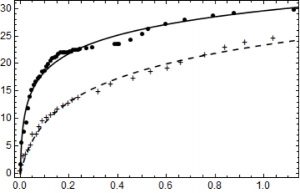
We ran simulations of Martian rocket plumes into craters that were much deeper than would really form, because the excessive depth only fills with stagnant pressure and does not affect the top of the simulation in steady state. This allows us to determine the length of a Martian plume in a crater, so we can determine how deep the crater will actually grow. We find that for human-class Mars missions, the crater can grow between 6 and 9 m deep. In reality, additional physics will probably prevent the crater from growing so deep, such as side-walls collapsing, sucked in by the Bernoulli effect of the coaxial jet. However, we can safely conclude that human-class Mars landers will create very deep holes that can be mission-threatening unless controlled. (Metzger and Li, 2018)
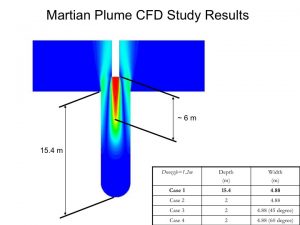
Diffusion Driven Shearing (DDS)
Our team’s research found that deep cratering is different if the soil is very porous, allowing gas pressure to build up under the surface faster than the pressure pushes it down into a crater, than if it is relatively impermeable so most of the gas pressure stays at the surface. In the latter case, what results is BCF. The former case we called Diffusion-Driven Shearing because the force is not applied at the surface of the soil anymore, but instead is distributed throughout the bulk of the soil. It is the result of the drag force of the permeating gas, pulling the soil everywhere and setting up elastic forces in the soil that exceed shear strength so the soil deforms plastically. In the BCF case, the sand moves perpendicularly away from the tip of the crater, but in the DDS case it moves tangentially around the tip. More generally, the soil has intermediate permeability so the gas is not fully diffused into the subsurface. Then, the force is applied partly at the surface due to stagnation pressure, and partly throughout the subsurface due to diffusing drag force. In those more general cases, the sand grains move diagonally away from the tip of the crater.
Our team observed the various cases from BCF through DDS, and we developed the theory of the DDS regime and performed computer simulations of it. (Metzger et al., 2009a)
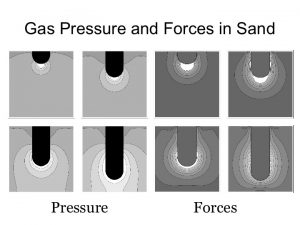
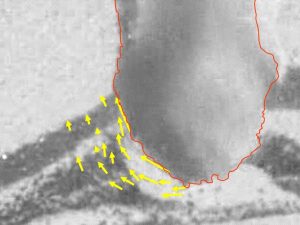
Diffused Gas Explosive Erosion (DGEE)
In studying the Mars Phoenix Lander plume effects, Anita Sengupta (NASA/JPL) and our team member Manish Mehta (NASA/MSFC) discovered a new regime in plume/soil interactions. The Phoenix propulsion system used pulsed engines, and in the scaled experiments the pulsing caused repeated shock waves to propagate into and through the subsurface of the soil. This provided kinetic energy as well as pressure gradients into the subsurface, causing the soil to lose shear strength so it was easily swept away by the plume, forming a deep crater. They developed a theory of DGEE based on a suite of experiments. (Mehta et al., 2011)
Bibliography
Our team members are listed in bold.
Anand, Anshu, Kyle J. Berger, Philip T. Metzger, and Christine M Hrenya, “Role of Collisions in Erosion of Regolith during a Lunar Landing,” Physical Review E87, 022205 (2013).
Beaty, D.W., Snook, K., Allen, C.C., Eppler, D., Farrell, W.M., Heldmann, J., Metzger, P., Peach, L., Wagner, S.A., and Zeitlin, C., “An Analysis of the Precursor Measurements of Mars Needed to Reduce the Risk of the First Human Missions to Mars,” unpublished report of the Mars Exploration Program Analysis Group (MEPAG), http://mepag.jpl.nasa.gov/reports/index.html(2005).
Berger, Kyle J., Anshu Anand, Philip T. Metzger, and Christine M Hrenya,, “Erratum: Role of collisions in erosion of regolith during a lunar landing (vol 87, 022205, 2013)”, Physical Review E91(1), 1-1 (2015).
Chambers, Wesley A., and Philip T. Metzger, “Regolith Instability Caused by Gas Diffusion: A Case Study of the Asteroid Redirect Mission,” Proceedings of Earth and Space 2016: Engineering, Science, Construction, and Operations in Challenging Environments, Orlando, FL, April 11-15, 2016.
Chambers, Wesley Allen, Philip Metzger, Adrienne Dove, and Daniel Britt. “Simulating regolith ejecta due to gas impingement.” In AAS/Division for Planetary Sciences Meeting Abstracts, vol. 48. 2016.
Clegg, Ryan N., Bradley L. Jolliff, and Philip T. Metzger, “Photometric Analysis of the Apollo Landing Sites,” Proceedings of Earth and Space 2012: Engineering, Science, Construction, and Operations in Challenging Environments, Pasadena, CA, Apr. 15-18, 2012.
Clegg, Ryan, Philip Metzger, Luke Roberson, and Stephen Huff, “Lunar Soil Erosion Physics for Landing Rockets on the Moon,” abstract #P12.013 in APS March Meeting 2010, March 15-19,2010,
Culbert, Chris, Diane Linne, Faith Chandler, Leslie Alexander, Sharon Jefferies, Kriss J Kennedy, Mark Lupisella, Phil Metzger, Nathan Moore, and Karen Taminger, NASA Technology Roadmap TA-04, Human Exploration Destination Systems, NASA, July 2015.
Amara L. Graps, JL Galache, Vishnu Reddy, Martin Elvis, Andy Rivkien, Paolo Tanga, Migo Mueller, Philippe Blondel, Patrick Michel, Henry Hsieh, Petr Pravec, Alex Parker, Pauli Laine, Philip Metzger, Daniel Britt, Philipp Maier, Bill Hartmann, Jan Thimo Grundmann, “Questions from the Asteroid Miners,” Asteroid Science Intersections with In-Space Mine Engineering 2016, Luxembourg City, 21-22 September 2016.
Immer, Christopher D., John E. Lane, Philip T. Metzger, and Sandra Clements,“Apollo Video Photogrammetry Estimation of Plume Impingement Effects,” Proceedings of Earth and Space 2008, 11th Biennial ASCE Aerospace Division International Conference on Engineering, Construction and Operations in Challenging Environments, Long Beach, California, Mar. 3-5, 2008.
Immer, Christopher D., John E. Lane, Philip T. Metzger, and Sandra Clements,“Apollo Video Photogrammetry Estimation of Plume Impingement Effects,” (July, 2011).
Immer, Christopher, Philip Metzger, “Rocket Cratering in Simulated Lunar and Martian Environments,” Proceedings of Earth and Space 2010, 12th Biennial ASCE Aerospace Division International Conference on Engineering, Construction and Operations in Challenging Environments, Honolulu, HI, Mar. 14-17, 2010.
Immer, Christopher D., Philip Metzger, Paul Hintze, Andrew Nick and Ryan Horan, “Apollo 12 Lunar Module Exhaust Plume Impingement on Lunar Surveyor III,” Icarus211(2), 1089-1102 (February 2011).
LaMarche, Casey Q., Philip T. Metzger, and Jennifer S. Curtis, “Cratering of a Lunar Soil Simulant, JSC-1A, by a Turbulent Subsonic Jet”, Proceedings of Earth and Space 2010, 12th Biennial ASCE Aerospace Division International Conference on Engineering, Construction and Operations in Challenging Environments, Honolulu, HI, Mar. 14-17, 2010.
LaMarche, Casey Q., Jennifer S. Curtis, and Philip T. Metzger (2010), “Permeability of JSC-1A: A Lunar Soil Simulant,” Icarus212 (1), 383-389 (March 2011). doi:10.1016/j.icarus.2010.12.015.
Lane, John, “Ground Camera Photogrammetry – 3D Debris Trajectory Analysis”, FY03 CTC Project Final Report,ASRC Aerospace, Kennedy Space Center, FL, April 9, 2004.
Lane, John E. “Perimeter Fence Study: High-Speed Film Camera Analysis of FOD Impacting Perimeter Fence,” unpublished presentation (ASRC-Aerospace, Kennedy Space Center, FL, 2004).
Lane, John, James Mantovani, Robert Mueller, M. Nugent, A. Nick, J. Schuler, and I. Townsend. “Optical Extinction Measurements of Dust Density in the GMRO Regolith Test Bin.” (2016).
Lane, John E., and Philip T. Metzger (2012), “Ballistics Model for Particles on a Horizontal Plane in a Vacuum Propelled by a Vertically Impinging Gas Jet,” Particulate Science and Technology30 (2), 196-208.
Lane, John E. and Philip T. Metzger, “Estimation of Apollo lunar dust transport using optical extinction measurements,” Acta Geophysica 63 (2), 568-599 (2014).
Lane, J.E., and Philip T. Metzger, “Image Analysis Based Estimates of Regolith Erosion Due to Plume Impingement Effect,” Proceedings of Earth and Space 2014: Engineering, Science, Construction, and Operations in Challenging Environments, St. Louis, MO, Oct. 27-29, 2014.
Lane, John E., Philip T. Metzger, and Christopher D. Immer, “Lagrangian trajectory modeling of lunar dust particles,” Proceedings of Earth and Space 2008, 11th Biennial ASCE Aerospace Division International Conference on Engineering, Construction and Operations in Challenging Environments, Long Beach, California, Mar. 3-5, 2008.
Lane, J. E., P.T. Metzger, and J.W. Carlson, “Lunar Dust Particles Blown By Lander Engine Exhaust in Rarefied and Compressible Flow,” Proceedings of Earth and Space 2010, 12th Biennial ASCE Aerospace Division International Conference on Engineering, Construction and Operations in Challenging Environments, Honolulu, HI, Mar. 14-17, 2010.
Lane, John, Steven Trigwell, Paul Hintze, and Philip Metzger, “Further Analysis on the Mystery of the Surveyor III Dust Deposits,” Proceedings of Earth and Space 2012: Engineering, Science, Construction, and Operations in Challenging Environments, Pasadena, CA, Apr. 15-18, 2012.
Mehta, Manish. “Plume-Surface Interactions due to Spacecraft Landings and The Discovery of Water on Mars.” PhD dissertation, University of Michigan, 2010.
Mehta, Manish, Nilton Renno, Aline Cotel, and Myron Grover. “Characterization of the impingement dynamics of pulsed rocket plumes with the ground at low ambient pressure.” In 43rd AIAA/ASME/SAE/ASEE Joint Propulsion Conference & Exhibit, p. 5707. 2007.
Mehta, Manish, Nilton O. Renno, Peter G. Huseman, Douglas S. Gulick, and Aline Cotel. “The interaction dynamics of pulsed retro-rocket plumes with the ground during spacecraft descent on Mars.” In International Planetary Probe Workshop. 2006.
Mehta, Manish, Nilton O. Renno, John Marshall, M. Rob Grover, Anita Sengupta, Neal A. Rusche, Jasper F. Kok, Raymond E. Arvidson, Wojciech J. Markiewicz, Mark T. Lemmon and Peter H. Smith, “Explosive erosion during the Phoenix landing exposes subsurface water on Mars,” Icarus, Vol. 211, No. 1, 2011, Pp. 172-194.
Mehta, Manish, Anita Sengupta, Mark Pokora, Leslie Hall, and Nilton Renno. “Mars Landing Engine Plume Impingement Ground Interaction.” In Earth and Space 2010: Engineering, Science, Construction, and Operations in Challenging Environments, pp. 143-157. 2010.
Mehta, Manish, Anita Sengupta, Nilton O. Renno, J. W. Norman, and D. S. Gulick. “Underexpanded Supersonic Plume Surface Interactions: Applications for Spacecraft Landings on Planetary Bodies.” (2011).
Mehta, Manish, Anita Sengupta, Nilton O. Renno, John W. Van Norman, Peter G. Huseman, Douglas S. Gulick, and Mark Pokora. “Thruster plume surface interactions: Applications for spacecraft landings on planetary bodies.” AIAA journal 51, no. 12 (2013): 2800-2818.
Metzger, Philip T., “Gas Diffusion through Coarse Regolith During Asteroid Sampling Operations,” Proceedings of Earth and Space 2014, 13th Biennial ASCE Aerospace Division International Conference on Engineering, Construction and Operations in Challenging Environments, St. Louis, California, Oct. 27-29, 2012.
Metzger, P.T., C.D. Immer, C.M. Donahue, B.T. Vu, R.C. Latta, III, M. Deyo-Svendsen., “Jet-induced cratering of a granular surface with application to lunar spaceports,” J. Aerospace Engineering22 (1),pp. 24-32 (January, 2009).
Metzger, Philip T., Christopher D. Immer, John E. Lane, Jacob Smith, Joseph W. Studak, and Brian F. Banker, “Rocket plume impingement on Mauna Kea lunar analog site compared with photogrammetry of Apollo lunar landing videos,” extended abstract for the First Joint Meeting of the Space Resources Roundtable and the Planetary and Terrestrial Mining Sciences Symposium (PTMSS), Golden , CO, June 8-10, 2010.
Metzger, Philip T., et al., “Prediction and Mitigation of Plume-Induced Spray of Soil during Lunar Landings,” presentation to NASA Lunar Architecture Team (August 2006).
Metzger, Philip T., “Estimation of Regolith Backscatter during OSIRIS-REx Sample Capture,” NASA report to OSIRIS-REx Project (2014).
Metzger, Philip T., “Plume Interactions with Asteroid Regolith during Proximity Operations,” NASA report to Asteroid Return Mission (ARM) Alternative Study (October 2014).
Metzger, Philip T., “Rocket Exhaust Blowing Soil in Near Vacuum Conditions is Faster than Predicted by Continuum Scaling Laws,” Proceedings of Earth and Space 2016: Engineering, Science, Construction, and Operations in Challenging Environments, Orlando, FL, April 11-15, 2016.
Metzger, P.T., C. D. Immer, J.E. Lane, J. Smith, J. W. Studak, and B. F. Banker, “Rocket plume impingment on Mauna Kea lunar analog site compared with photogrammetry of Apollo lunar landing videos,” extended abstract for the First Joint Meeting of the Space Resources Roundtable and the Planetary and Terrestrial Mining Sciences Symposium (PTMSS), Golden , CO, June 8-10, 2010.
Metzger, Philip T., John E. Lane, “Effects of Spacecraft Landings on the Moon,” 44th Lunar and Planetary Science Conference, Abstract #3093 (2013).
Metzger, Philip T., John E. Lane, and Christopher D. Immer, “Modification of Roberts’ theory for rocket exhaust plumes eroding lunar soil,” Proceedings of Earth and Space 2008, 11th Biennial ASCE Aerospace Division International Conference on Engineering, Construction and Operations in Challenging Environments, Long Beach, California, Mar. 3-5, 2008.
Metzger, Philip T., John E. Lane, Christopher D. Immer, and Sandra Clements, “Cratering and Blowing Soil by Rocket Engines During Lunar Landings,” Proceedings of the 6th International Conference on Case Histories in Geotechnical Engineering, Arlington, Virginia, Aug. 11-16, 2008.
Metzger, Philip T., John E. Lane, Christopher D. Immer, and Sandra Clements, “Cratering and Blowing Soil by Rocket Engines During Lunar Landings,” in Lunar Settlements,ed. by Haym Benaroya (CRC Press, 2010), pp. 551-576.
Metzger, P.T., John E. Lane, C.D. Immer, J.N. Gamsky, W. Hauslein, Xiaoyi Li, R.C. Latta III, C.M. Donahue, “Scaling of Erosion Rate in Subsonic Jet Experiments and Apollo Lunar Module Landings,” Proceedings of Earth and Space 2010, 12th Biennial ASCE Aerospace Division International Conference on Engineering, Construction and Operations in Challenging Environments, Honolulu, HI, Mar. 14-17, 2010.
Metzger, P.T., John E. Lane, Robert A. Carilli, Jason M. Long, and Kathy L. Shawn, “Photogrammetry and ballistic analysis of a high-flying projectile in the STS-124 space shuttle launch,” Acta Astronautica67, 217-229 (2010).
Metzger, Philip T., Robert C. Latta III, Jason M. Schuler, and Christopher D. Immer, “Craters Formed in Granular Beds by Impinging Jets of Gas,” Powders & Grains 2009 conference, Golden, Colorado, July 13-17, 2009.
Metzger, Philip T., and Xiaoyi Li, “Analysis of Mars Landing Crater Depth and Blast Ejecta Distances,” manuscript in preparation, 2018.
Metzger, Philip T., Xiaoyi Li, Christopher D. Immer, and John E. Lane, “ISRU Implications of Lunar and Martian Plume Effects,” AIAA 2009-1204, Proceedings of 47th AIAA Aerospace Sciences Conference, Orlando, FL, January 5-8, 2009.
Metzger, Philip T., and Robert P. Mueller, “Landing Plume Effects,” in Mars Design Reference Architecture 5.0, Addendum, NASA SP-2009-566-ADD, pp. 234-248 (2009).
Metzger, Philip T., Jacob Smith, and John E. Lane, “Phenomenology of Soil Erosion by Rocket Exhaust on the Moon and the Mauna Kea Lunar Analog Site,” Journal of Geophysical Research – Planets 116, E06005 (June 2011).
Metzger, P.T., B.T. Vu, D.E. Taylor, M.J. Kromann, M.Fuchs, B.Yurko, A.Dokos, C.D. Immer, J.E. Lane, M.B. Dunkel, C.M. Donahue, and R.C. Latta, III, “Cratering of Soil by Impinging Jets of Gas, with Application to Landing Rockets on Planetary Surfaces,” Proceedings of the 18th Engineering Mechanics Division Conference (Blacksburg, Virginia), June 3-6, 2007.
Morris, B., D. B. Goldstein, P. L. Varghese, and L. M. Trafton, “Plume Impingement on a Dusty Lunar Surface,” to appear in Rarefied Gas Dynamics, proceeding of the 27th International Symposium on Rarefied Gas Dynamics, Pacific Grove, CA., July, 2010.
Morris, A. B., D. B. Goldstein, P. L. Varghese, and L. M. Trafton. “Plume impingement on a dusty lunar surface.” In AIP Conference Proceedings, vol. 1333, no. 1, pp. 1187-1192. AIP, 2011.
Morris, A. B., D. B. Goldstein, P. L. Varghese, and L. M. Trafton. “Modeling the interaction between a rocket plume, scoured regolith, and a plume deflection fence.” In Earth and Space 2012: Engineering, Science, Construction, and Operations in Challenging Environments, pp. 189-198. 2012.
Morris, A. B., D. B. Goldstein, P. L. Varghese, and L. M. Trafton. “Far field deposition of scoured regolith resulting from lunar landings.” In AIP Conference Proceedings, vol. 1501, no. 1, pp. 1220-1227. AIP, 2012.
Morris, Aaron B., David B. Goldstein, Philip L. Varghese, and Laurence M. Trafton. “Approach for modeling rocket plume impingement and dust dispersal on the moon.” Journal of Spacecraft and Rockets 52, no. 2 (2015): 362-374.
Morris, Aaron B., David B. Goldstein, Philip L. Varghese, and Laurence M. Trafton. “Lunar Dust Transport Resulting from Single-and Four-Engine Plume Impingement.” AIAA Journal vol. 54, no. 4 (2015): 1339-1349.
Mueller, R. P., L. Sibille, K. Leucht, J. D. Smith, I. I. Townsend, A. J. Nick, and J. M. Schuler. “Swamp Works: A New Approach to Develop Space Mining and Resource Extraction Technologies at the National Aeronautics Space Administration (NASA) Kennedy Space Center (KSC).” (2015).
Murray, J. A., S. Benyahia , P. Metzger, and C. M. Hrenya, “Continuum Representation of a Continuous Size Distribution of Particles engaged in Rapid Granular Flow,” Physics of Fluids24, 083303 (2012).
NASA, NASA’s Recommendations to Space-Faring Entities: How to Protect and Preserve the Historic and Scientific Value of U.S. Government Lunar Artifacts, https://www.nasa.gov/sites/default/files/617743main_NASA-USG_LUNAR_HISTORIC_SITES_RevA-508.pdf
Plemmons, D. H., M. Mehta, B. C. Clark, S. P. Kounaves, L. L. Peach, N. O. Renno, L. Tamppari, and S. M. M. Young. “Effects of the Phoenix Lander descent thruster plume on the Martian surface.” Journal of Geophysical Research: Planets113, no. E3 (2008).
Sengupta, Anita, James Kulleck, John Van Norman, and Manish Mehta. “Thermal coating erosion in a simulated Martian landing environment.” Wear 270, no. 5-6 (2011): 335-343.
Sengupta, Anita, James Kulleck, Steve Sell, John Van Norman, Manish Mehta, and Mark Pokora. “Mars lander engine plume impingement environment of the Mars Science Laboratory.” In Aerospace conference, 2009 IEEE, pp. 1-10. IEEE, 2009.
Sengupta, A., M. Mehta, J. Vizcaino, and P. Metzger, “Plume Impingement Induced Surface Erosion During Retro-Propulsive Landings on Mars”, in 11th International Planetary Probe Workshop (June 16–20, 2014 in Pasadena, California), abstract no. 8118.
Shipley, S.T., P.T. Metzger and J.E. Lane, “Lunar Cold Trap Contamination by Landing Vehicles,” Proceedings of Earth and Space 2014: Engineering, Science, Construction, and Operations in Challenging Environments, St. Louis, MO, Oct. 27-29, 2014.
Vizcaino, Jeffrey, and Manish Mehta. “Quantification of plume-soil interaction and excavation due to the Mars Science Laboratory Sky Crane Descent Phase”, 8th Symposium on Space Resource Utilization, AIAA SciTech Forum, (AIAA 2015-1649)
Wittbrodt, Audelia C., and Philip T. Metzger. “Material Damage from Impacts of Lunar Soil Particles Ejected by the Rocket Exhaust of Landing Spacecraft.” NASA Technical Report KSC-2008-290 (2008).
Zacny, Kris, Edward B. Bierhaus, Daniel T. Britt, Benton Clark, Christine M. Hartzell, Leslie Gertsch, Anton V. Kulchitsky, Anton V., Johnson, Jerome. B., Metzger, Phil, Reeves, David M. and Sanchez, Paul. “Geotechnical Properties of Asteroids Affecting Surface Operations, Mining, and In Situ Resource Utilization Activities.” In Primitive Meteorites and Asteroids, pp. 439-476. Elsevier, 2018.
Next: Geological Considerations for Plume Effects
Back: Why Planetary Landing Plume Effects Are Important
<< Return to Main Page